Is there a fundamental logic to life?
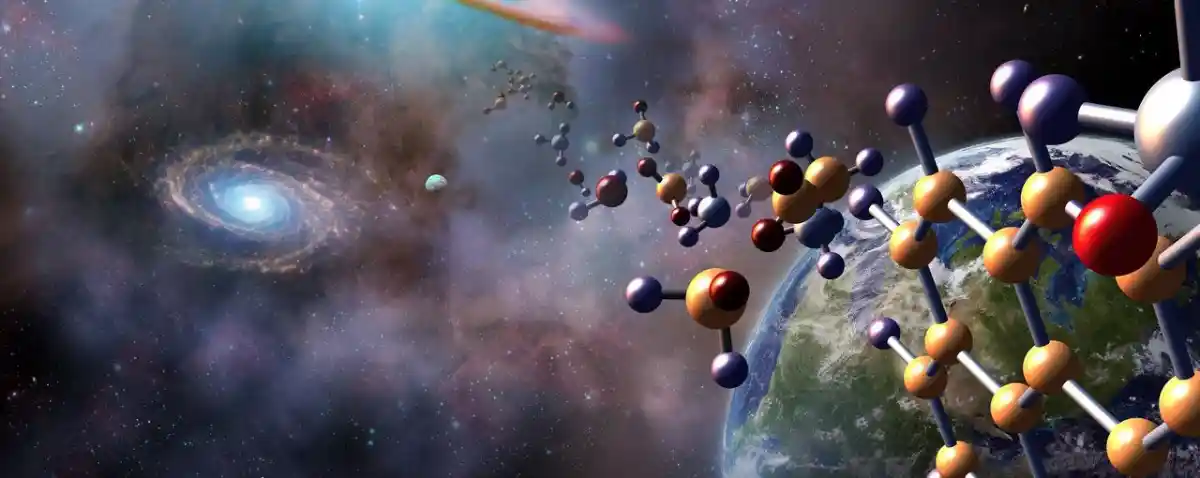
The search for extraterrestrial life raises profound questions, and one of the most challenging is defining the nature of life itself. For over a century, biologists have understood that life on Earth is rooted in fundamental building blocks: DNA, RNA, and amino acids. Fossil records reveal that life’s evolution has followed diverse pathways, leading to the incredible variety of organisms we see today. Yet, patterns of convergence and constraints suggest that evolutionary possibilities are not infinite, limiting the forms life can take.
For astrobiologists, these insights spark compelling questions about life beyond Earth. Can we predict the nature of alien life based on our terrestrial framework? How much do the constraints observed on Earth apply elsewhere? A recent study led by an international team of researchers at the Santa Fe Institute (SFI) explored these issues.
Drawing from case studies across multiple disciplines, they concluded that fundamental limits exist, restricting the kinds of life forms that could emerge anywhere in the cosmos.
The research team was led by Ricard Solé, the head of the ICREA-Complex Systems Lab at the Universitat Pompeu Fabra and an External Professor at the Santa Fe Institute (SFI). He was joined by multiple SFI colleagues and researchers from the Institute of Biology at the University of Graz, the Complex Multilayer Networks Lab, the Padua Center for Network Medicine (PCNM), Umeå University, the Massachusetts Institute of Technology (MIT), the Georgia Institute of Technology, the Tokyo Institute of Technology, and the European Centre for Living Technology (ECLT).

The team explored the potential findings of an interstellar probe landing on an exoplanet in search of life. A critical question arose: how could such a mission identify life forms that evolved in entirely different biospheres from Earth’s? While life likely requires certain physical and chemical preconditions to arise—improving the odds of detection—identifying it becomes significantly more complex when considering synthetic biology and bioengineering alongside evolutionary biology and astrobiology.
As Solé and his team noted, these complexities boil down to a fundamental question: can scientists anticipate the range of possible living systems beyond Earth’s biosphere? Solé highlighted two key challenges: the uncertainty about what to search for and the impact of synthetic biology, which complicates astrobiological studies.
“The big issue is the detection of biosignatures. Detecting exoplanet atmospheres with the proper resolution is becoming a reality and will improve over the following decades. But how do we define a solid criterion to say that a measured chemical composition is connected to life?
“[Synthetic biology] will be a parallel thread in this adventure. Synthetic life can provide profound clues on what to expect and how likely it is under given conditions. To us, synthetic biology is a powerful way to interrogate nature about the possible.”

To address these foundational questions, the team examined case studies spanning thermodynamics, computation, genetics, cellular development, brain science, ecology, and evolution.
They also reviewed prior research modeling evolution through concepts like convergent evolution (independent evolution of similar traits or behaviors), natural selection, and the constraints of a biosphere. From these analyses, Solé and his team identified universal characteristics that all life forms seem to share:
“We have looked at the most fundamental level: the logic of life across sales, given several informational, physical, and chemical boundaries that seem to be inescapable. Cells as fundamental units, for example, seem to be an expected attractor in terms of structure: vesicles and micelles are automatically formed and allow for the emergence of discrete units.”
The team also drew on historical examples where theoretical predictions about life’s complexity were later validated by biology. A notable case is Erwin Schrödinger’s 1944 book What is Life?, in which he proposed that genetic material is an aperiodic crystal—a non-repeating yet precisely ordered structure capable of encoding the information needed to guide an organism’s development.
Schrödinger’s insights directly influenced James Watson and Francis Crick, leading to their discovery of DNA’s structure in 1953.
Additionally, Solé highlighted the forward-thinking contributions of John von Neumann, whose “universal constructor” concept predated the molecular biology revolution. Von Neumann’s theoretical model of a self-replicating machine drew inspiration from the logic underpinning cellular life and reproduction.
“Life could, in principle, adopt a wide array of configurations,” Solé noted. “However, we argue that certain features are unavoidable across all life forms—such as linear information polymers and the existence of parasitic systems.”

In the meantime, Solé emphasized that much work remains before astrobiology can reliably predict the potential forms life might take across the Universe:
“We propose a set of case studies that cover a broad range of life complexity properties. This provides a well-defined road map to developing the fundamentals. In some cases, such as the inevitability of parasites, the observation is enormously strong, and we have some intuitions about why this happens, but not yet a theoretical argument that is universal. Developing and proving these ideas will require novel connections among diverse fields, from computation and synthetic biology to ecology and evolution.”
The team’s paper, “Fundamental constraints to the logic of living systems,” appeared in Interface Focus (a Royal Society publication).
Further Reading: Santa Fe Institute, Interface Focus