A recently published study posits that the black holes postulated by Einstein’s general theory of relativity may not be as featureless and structureless as previously thought. Alternatively, these cosmic entities may be constituted by quantum objects designated as “frozen stars.”
Although these hypothetical celestial bodies would share some similarities with black holes, they differ in crucial ways that could potentially resolve the infamous Hawking radiation paradox, named for the late physicist Stephen Hawking, who proposed the phenomenon. This paradox arises because the theoretical radiation emitted by a black hole’s event horizon seems to carry no information about the matter that formed the black hole. This is in contradiction with a fundamental principle of quantum mechanics, namely that information cannot be destroyed.
Furthermore, in contrast to the conventional black holes, the frozen stars are not anticipated to possess a singularity—a point of infinite density at their cores—which resolves another discrepancy between the classical conception of black holes and the fundamental tenet in physics that infinities cannot exist in nature. When infinities emerge in a theory, it typically indicates the theory’s limitations.
“Frozen stars are a type of black hole mimickers: ultracompact, astrophysical objects that are free of singularities, lack a horizon, but yet can mimic all of the observable properties of black holes,” Ramy Brustein, a professor of physics at Ben-Gurion University in Israel, told Live Science in an email. “If they actually exist, they would indicate the need to modify in a significant and fundamental way Einstein’s theory of general relativity.”
Brustein is the principal author of a study delineating the frozen star theory, which was published in July in the journal Physical Review D.
The classical model of a black hole, initially delineated by Karl Schwarzschild in 1916, depicts black holes as possessing two pivotal characteristics: a singularity, wherein the entirety of the mass is concentrated, and an event horizon, a boundary from which nothing, not even light, can evade.
However, this model encounters a significant challenge when quantum mechanics is introduced. In the 1970s, Stephen Hawking famously demonstrated that quantum effects near the event horizon should result in the creation of particles from the vacuum of space, a process known as Hawking radiation. This radiation would cause the black hole to gradually lose mass and eventually evaporate completely.
The paradox arises from the observation that this radiation appears to carry no information about the matter from which the black hole was formed. If the black hole evaporates completely, this information is effectively lost, which is in violation of the principles of quantum mechanics, which dictate that information must be conserved. This contradiction is referred to as the information loss paradox, representing a significant challenge in the field of theoretical physics.
In their new study, Brustein and his co-authors A.J.M. Medved of Rhodes University and Tamar Simhon of Ben-Gurion University conducted a comprehensive theoretical examination of the frozen stars model. Their findings indicate that this model effectively addresses the paradoxes inherent to the traditional model, as it lacks both a horizon and a singularity.
The authors discovered that if black holes are, in fact, extremely compact entities comprising ultra-rigid matter whose properties are informed by string theory, the foremost candidate for the theory of quantum gravity, they do not collapse into infinitely dense points and possess a size slightly larger than the conventional event horizon, thereby preventing the latter from forming.
“We have shown how frozen stars behave as (nearly) perfect absorbers although lacking a horizon and act as a source of gravitational waves,” said Brustein, noting that these objects can absorb almost everything that falls onto them, much like black holes. “Moreover, they source the same external geometry as that of a conventional model of black holes and reproduce their conventional thermodynamic properties.”
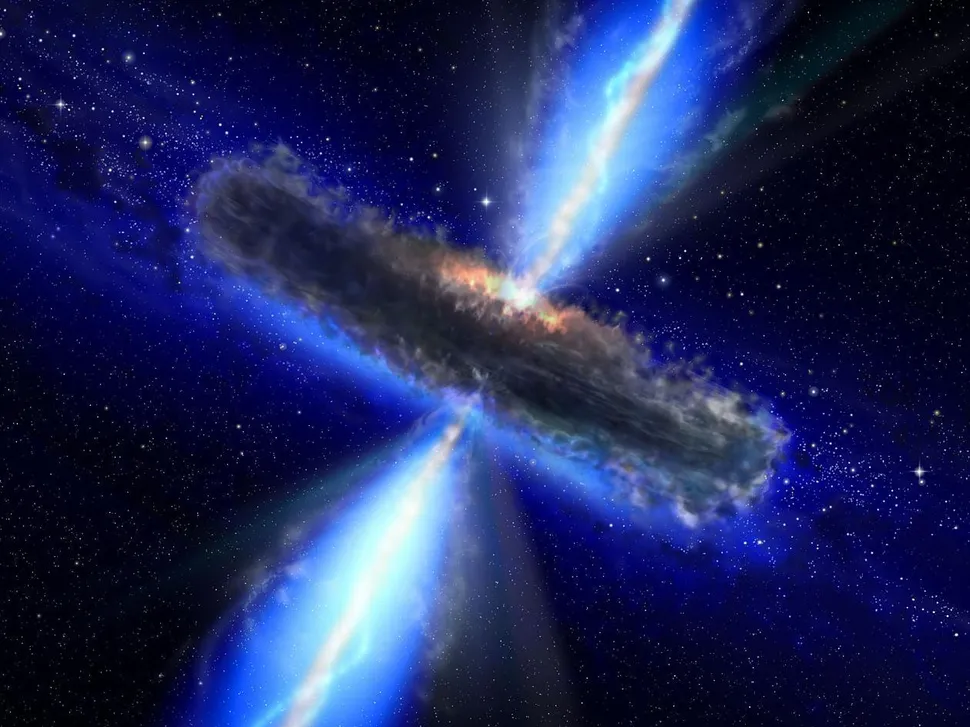
Although the frozen star model offers a promising avenue for understanding the paradoxes associated with traditional black holes, further experimental verification is necessary.
However, in contrast to conventional black holes, frozen stars are anticipated to possess an internal structure, albeit one exhibiting unconventional properties that are a consequence of quantum gravity. This paves the way for observational differentiation between the two. The evidence may be found in the gravitational waves—ripples in the fabric of spacetime—generated during black hole mergers.
“This is when the distinctions would be most pronounced,” explained Brustein.
The team must still determine the precise internal structure of a frozen star and how it differs from other extreme cosmic objects, such as neutron stars. However, Brustein asserted that this is a feasible goal. Subsequently, the data obtained from existing and future gravitational wave observatories could be subjected to analysis, as the gravitational waves emitted during the mergers are of an extremely powerful nature and are capable of conveying information regarding the structure of these ultracompact objects.